We use cookies to make your experience better. To comply with the new e-Privacy directive, we need to ask for your consent to set the cookies.
Microwave Synthesis Reactor Designs
Microwave instrumentation has evolved to include systems designed specifically to meet the needs of synthetic chemists.
The first microwave systems employed for laboratory applications were simple, inexpensive, domestic microwave ovens designed for home use. Creative chemists saw these devices as a cost-effective means to investigate the use of microwave energy to drive chemical reactions. These systems were commonly available, easy to operate, and due to their unsophisticated design, simple to modify for concept testing. For the most part, applications that required long heating cycles or had high temperature needs under conventional methods, such as sample preparation for trace analysis, were explored first.
Results from the use of microwave energy to promote these applications were often promising, but with safety and hardware limitations. Namely, these systems were not designed for the harsh conditions encountered in the laboratory, nor did they permit the kind of flexibility in reaction handling and software programming necessary to gain large scale use. The need for equipment specifically designed for the laboratory became more evident as experimentation with microwave-enhanced chemistry began to progress.
Multi-mode vs. Single-mode microwave cavity
The earliest attempts to design microwave-based laboratory instrumentation centered on the modification of domestic microwave ovens to enhance their ability to survive laboratory conditions. As such, multi-mode microwave applicators, the basic applicator used in domestic ovens, saw the earliest use in the laboratory. These applicators feature larger cavity geometries, which allow the processing of multiple samples simultaneously. Sample containers of various sizes and shapes from micro titer plates to large vessels, such as three L-flasks have been successfully used in multi-mode systems.
Multi-mode cavities, due to the physics of their design, have multiple pockets of energy dispersed throughout the cavity volume. Multiple energy pockets will have different levels of energy intensity, sometimes referred to as hot and cold spots. Therefore, any one sample may encounter an energy pocket of greater or lesser intensity than another if positioned statically in a multi-mode cavity. In order to provide an equal distribution of energy, multi-mode systems continuously rotate samples throughout the energy field. This tends to smooth or average the field exposure across all of the samples during the energy cycle.
For the organic chemist, microwave systems seem to provide an obvious resolution for the need to accelerate long, laborious reaction procedures. Medicinal chemists see microwave systems as a way to accelerate optimization protocols. However, it is the combinatorial chemist who greets the use of microwave chemistry most openly — as a way to increase reaction throughput in parallel chemistry applications. In all cases, the use of multi-mode microwave systems has proven to be of limited advantage.
For organic and medicinal chemists, the lack of field homogeneity paired with the positional sensitivity of these systems produce results that are at once promising and distressing. Often reactions will show great improvements only to have reproduction of the result elude the investigator. For the combinatorial chemist, the combination of field inhomogeneity coupled with the differences in sample absorption characteristics make the control of chemistries conducted simultaneously in parallel formats difficult when diverse chemistries are attempted. Specifically, multi-mode systems become problematic for chemists trying to reproduce their reactions on a small scale. While the total power generated may be high in industrial multi-mode instruments (typically in the 1000 – 1200 W range), the power density of the field is quite low due to the total volume of the cavity (typically in the 0.025 – 0.040 W/mL range). Therefore, trying to heat the small individual samples characteristic of drug discovery or new chemistry research is difficult.
To combat the inherent difficulty with multi-mode technology, instrumentation manufacturers developed single-mode cavities with more consistent and predictable energy distribution.13 Single-mode instruments produce one homogenous, intense pocket of energy that is highly reproducible. Due to their uniform energy distribution and higher power density, these systems typically couple more efficiently with small samples. Although single-mode systems only output 300 – 400 W of power, their smaller cavity volume and single, focused energy pocket yield a field density in the 0.90 W/mL range.
Single-mode systems were not widely used, however, until recent advances in technology led to the development of instruments with the necessary features and software to be of use in organic synthesis laboratories. Chemists wanted the ability to perform elevated pressure and atmospheric reactions. In addition, they needed a way to “tune” the instrument for particular applications, as changes in sample size or the physical coupling characteristics of the sample (i.e. polarity of the sample, conductive properties of the sample) can dramatically affect the ability of the applicator to couple with the sample. Only in the last few years have systems become available that either allow the user to tune the applicator or provide applicators that automatically tune to changing application needs.
The need to change the tuning of these cavities can be difficult if a chemist is processing several different types of samples concurrently. Figure 43 shows the side view of an older single-mode design. There is a rectangular waveguide (the microwave cavity), a power source, a sample positioned at a maximized energy point from the magnetron, and some type of mechanical tuning device in the system that will adjust it for variations in the sample.8,12,722,723
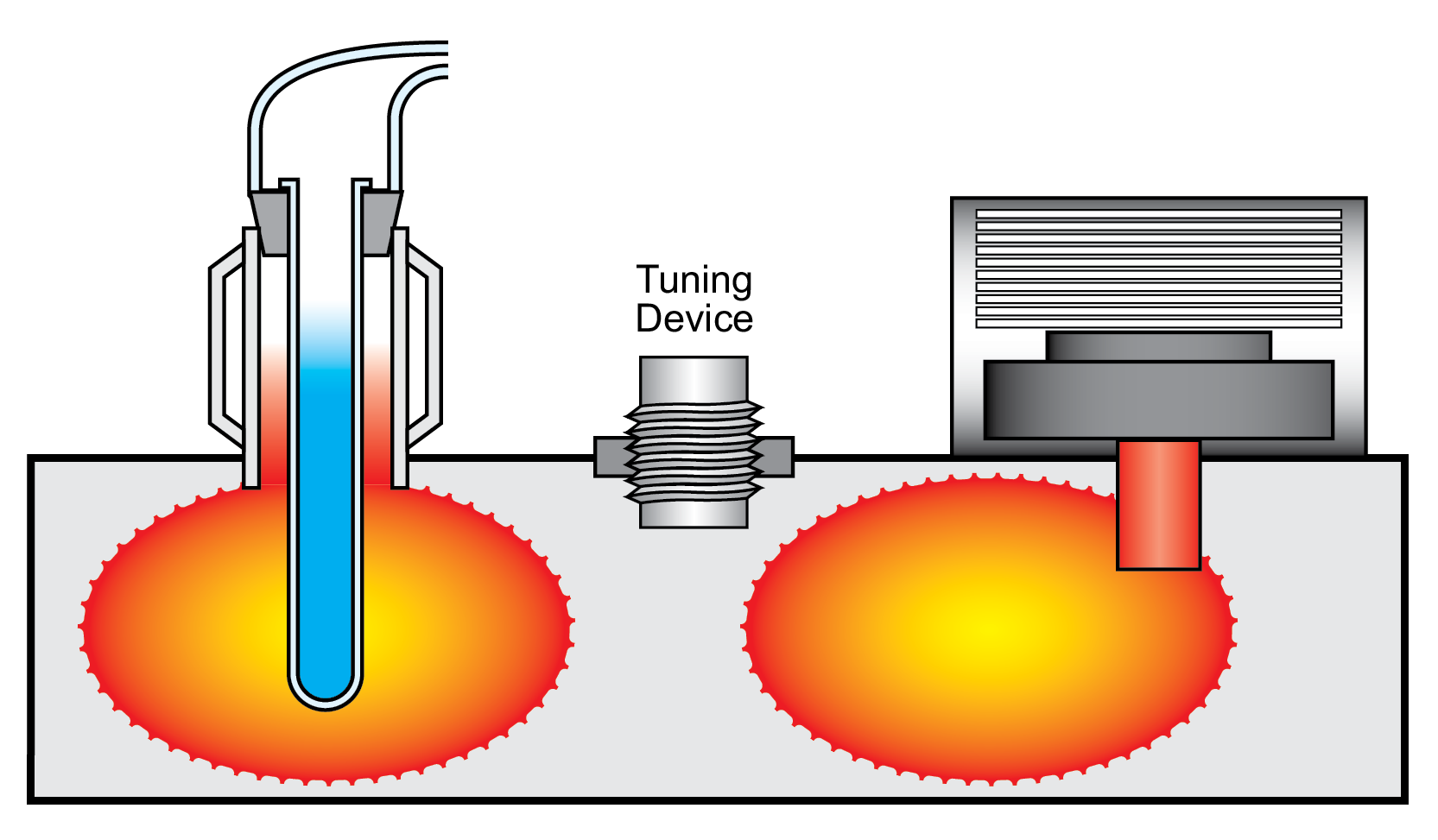
Figure 43: Single-mode microwave applicator
Due to recent advances in single-mode microwave technology, systems now offer greater flexibility to the organic chemist. In addition to the traditional rectangular waveguide style applicator, a circular waveguide capable of self-tuning is now available (Figure 44, top view). This applicator features multiple entry points for the microwave energy to enter the cavity, compensating for variations in the coupling characteristics of the sample, the physical size of the sample, and the geometrical placement of the sample in the cavity. This design feature effectively renders the cavity immune to tuning issues.
The circular waveguide design also equates to a larger volume than preceding single-mode designs, offering flexibility in terms of sample volume. It can accept sample containers ranging from 1 mL up to 125 mL in size. Using infrared thermometry technology, this system allows volumes as low as 100 μL in specially designed pressure tubes. Additionally, it can be used with open vessels for performing traditional atmospheric work. This provides an open system in which the chemist can add reagents to the sample while it refluxes. This technology has incorporated all of the desirable capabilities of conventional heating methods into a microwave system while also offering the benefits of greatly increased reaction times and improved yields.

Figure 44: Self-tuning single-mode microwave applicator
Sealed Vial vs. Open Vessel Reactions
Most single-mode applicators permit the use of sealed vials to perform elevated pressure reactions. As previously mentioned, the circular waveguide design can also perform reactions at atmospheric pressure. This applicator design employs two different sized attenuators (or doors), one to use with pressure tubes and another that will fit around the necks of round-bottom flasks. This configuration allows the operator to choose the glassware to fit a specific application need.
Most systems incorporate the use of 10-mL pressure vials and are preferably capped with self-sealing septa. These systems monitor the reaction conditions, and offer feedback control of the power input based on this monitored information. The available systems offer two pressure sensor design philosophies, direct and indirect measurement. Direct pressure measurement inserts a needle probe into the septum of the reaction tube. It has the benefit of fast response time, allowing a precise control of the reaction environment. The disadvantage to the direct method is that the pressure in the vessel must be lowered before the cover may be removed. This typically takes about one minute from the completion of a run. With the indirect method, the deflection of the septum is measured and related to the internal pressure of the vial. This is beneficial when working with highly corrosive reagents, as there is no septum penetration, but it does present some lag time in the measurement.
Using the circular waveguide design, atmospheric reactions can be done in standard round-bottom flasks up to 125 mL in size and with neck joint sizes of 24/40 and smaller. Reflux condensers, addition funnels, Dean-Stark traps, or any other glass apparatus that is needed can be used. Depending on the type of reaction you are doing and the temperatures that are needed, atmospheric conditions may be the method of choice. Microwave organic reactions performed under atmospheric conditions may not produce the enhancements seen with pressurized vessels, but they can give reaction rates that are 10x faster than conventional methods and have the advantage of offering chemists the opportunity to increase the scale of the reaction.
Cooling
Microwave irradiation can take reactions up to extremely high temperatures. Some single-mode systems include a cooling feature that allows for fast reaction quenching as compressed gas is forced into the reaction cavity. As the gas expands, it cools the atmosphere inside the cavity. The accelerated cooling profile reduces side reactions and provides for cleaner chemistries, resulting in less post-reaction workup (Figure 45).
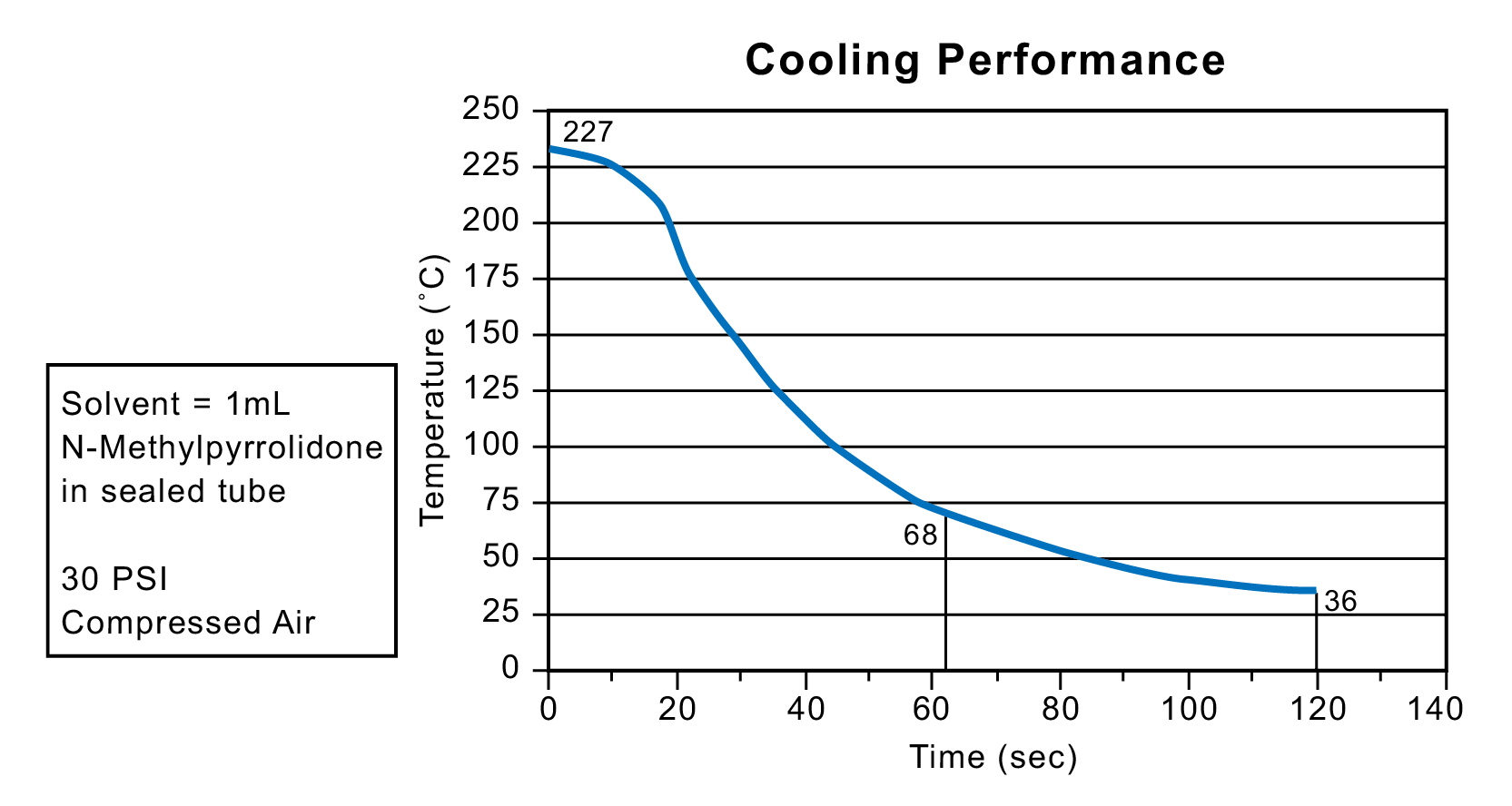
Figure 45: Cooling performance
Some systems also have the ability to activate the cooling feature during reactions to control bulk temperature rise. This can be especially useful in reactions where the reagents and/or products are heat sensitive. If compressed air is introduced into the cavity while simultaneously applying microwave irradiation, thermal heat will not accumulate in the reaction mixture. Large amounts of energy can still be applied while the bulk temperature remains low, resulting in higher product yields and cleaner chemistries for many reactions.
Automation
Some single-mode systems are automated for increased throughput and unattended operation. As the typical reaction times are short (2-10 minutes), an automated sequential procedure allows for total reaction control and optimization while approaching parallel throughput results. The practice of High Throughput Sequential Chemistry (HTSC) allows for the ultimate in reaction optimization as the individual parameters of each reaction may be modified during the process to promote the most advantageous result.
As opposed to traditional parallel synthesis, where the reaction conditions are held constant for a parallel batch of chemistries, HTSC offers the chemist complete control over every step of each reaction in an optimization procedure, a feat that was too time-consuming and labor-intensive to be realistically attainable before. Automated formats permit the best practice of HTSC, as an entire library may be generated unattended with completely different reaction protocols within the same timeframe as traditional parallel schemes. Increased diversity and control without limited throughput make automated systems a popular choice with many chemists.
Instruments
8. Loupy, A.; Petit, A.; Hamelin, J.; Texier-Boullet, F.; Jacquault, P.; Mathe, D. “New solvent-free organic synthesis using focused microwaves.” Synthesis 1998, 9, pp. 1213-34.
12. Johannsson, H. “A solution to the bottleneck in drug discovery.” Am. Laboratory 2001, 33, pp. 28-32.
13. Larhed, M.; Hallberg, A. “Microwave-assisted high-speed chemistry: a new technique in drug discovery.” Drug Discovery Today 2001, 6, pp. 406-16.
722. European Patent No. 0155893, “Appareil de reaction chimique par voie humide de produits divers.” 1985.
723. Gasgnier, M.; Loupy, A.; Petit, A.; Jullien, H. “New developments in the field of energy transfer by means of mono-mode microwaves for various oxides and hydroxides.” J. Alloys Compd. 1994, 204, pp. 165-72.